Antimatter is the same as ordinary matter except that it has the opposite electric charge. For instance, an electron, which has a negative charge, has an antimatter partner known as a positron. A positron is a particle with the same mass as an electron but a positive charge.
Particles with no electric charge, like neutrons, are often their own antimatter partners. But researchers have yet to determine if mysterious tiny particles known as neutrinos, which are also neutral, are their own antiparticles.
Although it may sound like something out of science fiction, antimatter is real. Antimatter was created along with matter after the Big Bang. But antimatter is rare in today's universe, and scientists aren't sure why.
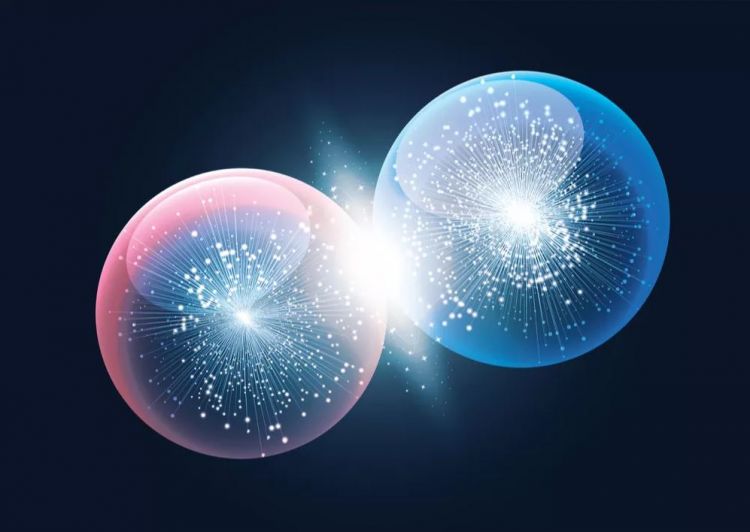
In physics, antimatter is defined as the opposite of matter, having the same mass as matter but opposite electric charge. For example, a positron is the antimatter equivalent of an electron. It has a mass equal to that of an electron, but the charge of a positron is +1 as compared to -1 of an electron.
For the past few years, scientists at CERN (European Organization for Nuclear Research) have been synthesizing antihydrogen by slowing down high-energy antiprotons and smashing them into positrons. But since antimatter is explosive (it blasts when it comes in contact with normal matter) and energy-intensive production, the cost of making antimatter is too high.
The cost of 1 gram of antimatter is about 62.5 trillion dollars (around 5,000 billion Indian rupees).
Matter and Antimatter
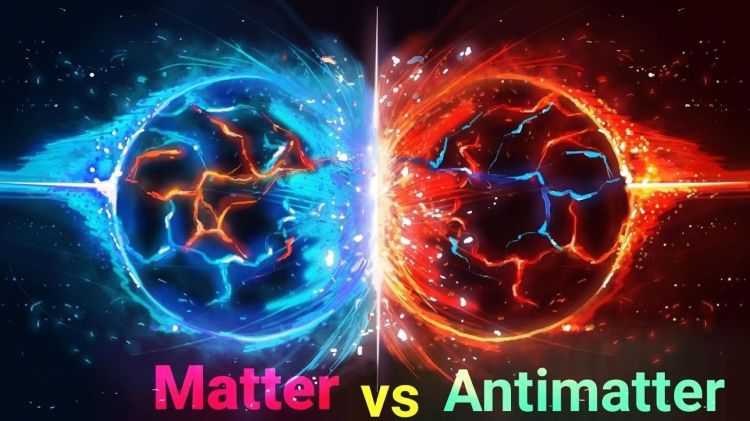
The positron was only the first example of antimatter. Every particle in nature has an antimatter counterpart, although some particles, like the photon, are their own antiparticles. Antimatter has charge opposite to that of matter (for example, the positron is positive while the electron is negative) but is nearly identical otherwise, having the same mass, intrinsic spin, half-life, and so on. When a particle and its antimatter counterpart interact, they annihilate one another, usually totally converting their masses to pure energy in the form of photons as seen in Figure 2. Neutral particles, such as neutrons, have neutral antimatter counterparts, which also annihilate when they interact. Certain neutral particles are their own antiparticle and live correspondingly short lives. For example, the neutral pion \boldsymbol{\pi ^0} is its own antiparticle and has a half-life about \boldsymbol{10^{-8}} shorter than \boldsymbol{\pi ^+} and \boldsymbol{\pi ^-}, which are each other’s antiparticles. Without exception, nature is symmetric—all particles have antimatter counterparts. For example, antiprotons and antineutrons were first created in accelerator experiments in 1956 and the antiproton is negative. Antihydrogen atoms, consisting of an antiproton and antielectron, were observed in 1995 at CERN, too. It is possible to contain large-scale antimatter particles such as antiprotons by using electromagnetic traps that confine the particles within a magnetic field so that they don’t annihilate with other particles. However, particles of the same charge repel each other, so the more particles that are contained in a trap, the more energy is needed to power the magnetic field that contains them. It is not currently possible to store a significant quantity of antiprotons. At any rate, we now see that negative charge is associated with both low-mass (electrons) and high-mass particles (antiprotons) and the apparent asymmetry is not there. But this knowledge does raise another question—why is there such a predominance of matter and so little antimatter? Possible explanations emerge later in this and the next chapter.
WHERE IS ANTIMATTER, AND HOW IS ANTIMATTER MADE?
Humans have created antimatter particles using ultra-high-speed collisions at huge particle accelerators such as the Large Hadron Collider, which is located outside Geneva and operated by CERN (the European Organization for Nuclear Research). Several experiments at CERN create antihydrogen, the antimatter twin of the element hydrogen. The most complex antimatter element produced to date is antihelium, the counterpart to helium.
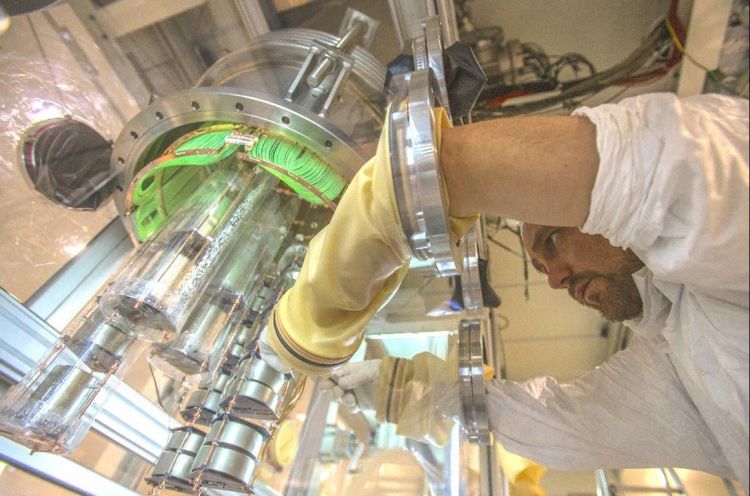
There are also naturally produced antiparticles made sporadically throughout the universe. But when matter and antimatter meet, they annihilate each other and produce energy, meaning that in a matter-dominated cosmos such as ours, antimatter doesn't stick around very long.
Antimatter is also at the heart of a mystery about why the universe exists at all. In the first moments after the Big Bang, only energy existed. As the universe cooled and expanded, particles of both matter and antimatter were produced. Scientists have measured the properties of particles and antiparticles with extremely high precision and found that both behave identically. So if antimatter and matter were created in equal amounts and they behave identically, all the matter and antimatter created at the beginning of time should have annihilated on contact, leaving nothing behind.
Why matter came to dominate over antimatter is a major mystery.
One theory suggests that more matter than antimatter was created in the beginning of the universe, so that even after mutual annihilation, there was enough matter left to form stars, galaxies and, eventually, everything on Earth. The discrepancy would have been very tiny. Less than 1 in 1 billion ordinary particles would have survived the chaos and gone on to form all the matter around us today, according to Space.com, a Live Science sister site.
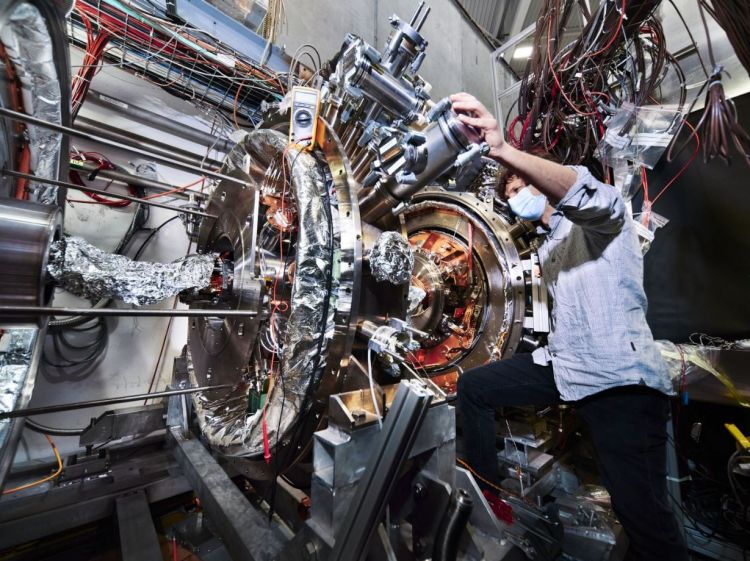
If the neutrino — a tiny, ghostly particle that barely interacts with other matter — actually is its own antiparticle, that might be the key to solving this problem. In this theory, at the start of time, a small fraction of neutrinos would have been able to transition from antimatter to matter, potentially creating a slight matter imbalance at the universe's inception. Experiments have tried to determine if the neutrino is its own antiparticle, but so far, they have turned up inconclusive.
PREDICTION AND NOBEL PRIZE
British physicist Paul Dirac predicted antimatter in 1928 while trying to combine quantum mechanics, which describes subatomic particles, and Einstein's theory of relativity. Dirac was looking at solutions to an equation that described the movement of an electron traveling near the speed of light. "Just as the equation x^2 = 4 can have two possible solutions (x = 2 or x = minus −2), so Dirac's equation could have two solutions, one for an electron with positive energy, and one for an electron with negative energy," according to CERN.
At first, Dirac was hesitant about sharing his findings. But eventually, he embraced them and said every particle in the universe should have a mirror-image particle that behaved like it but had an opposite charge.
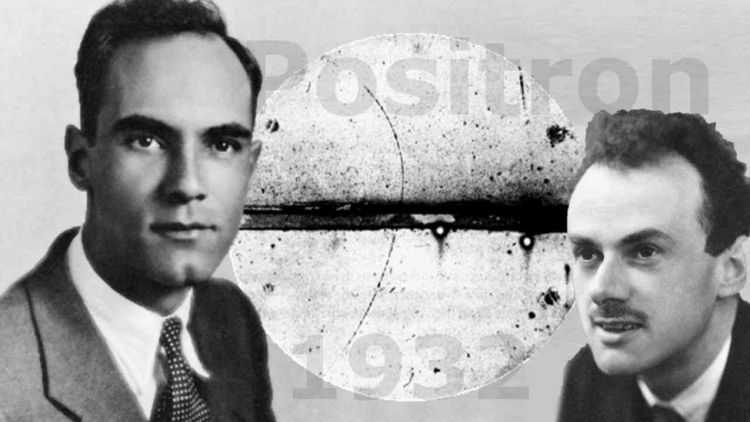
Positrons were discovered a few years later by American California Institute of Technology physicist Carl Anderson, who was studying highly energetic cosmic rays that come from space and hit Earth's atmosphere, producing a shower of other particles. In his detector, Anderson witnessed a trace of something with the same mass as an electron but with a positive charge. An editor at the journal Physical Review suggested the name positron for the particle, according to the American Institute of Physics.
For their work on this discovery, Dirac and Anderson received the Nobel Prize in physics — Dirac in 1933, and Anderson in 1936.
Also Read: What is Hyperloop and how can it Traveling speed over 1280 Kilometer /hour